Can bacteria be engineered in ways that they help our bodies better fight cancer?
That’s the goal Tal Danino, Ph.D., is pursuing as a Cancer Research Institute (CRI) Lloyd J. Old STAR, a program named after the “Father of Modern Tumor Immunology” who served as CRI’s founding scientific and medical director.
Trillions of bacteria naturally reside in and on our bodies. They perform important functions, and without them we wouldn’t survive. On the skin and in the gut, they help regulate the immune system and keep its activity balanced. With CRI support, Danino, an associate professor in Columbia University’s department of biomedical engineering, is now exploring how we might boost their benefits even more, especially against cancer.
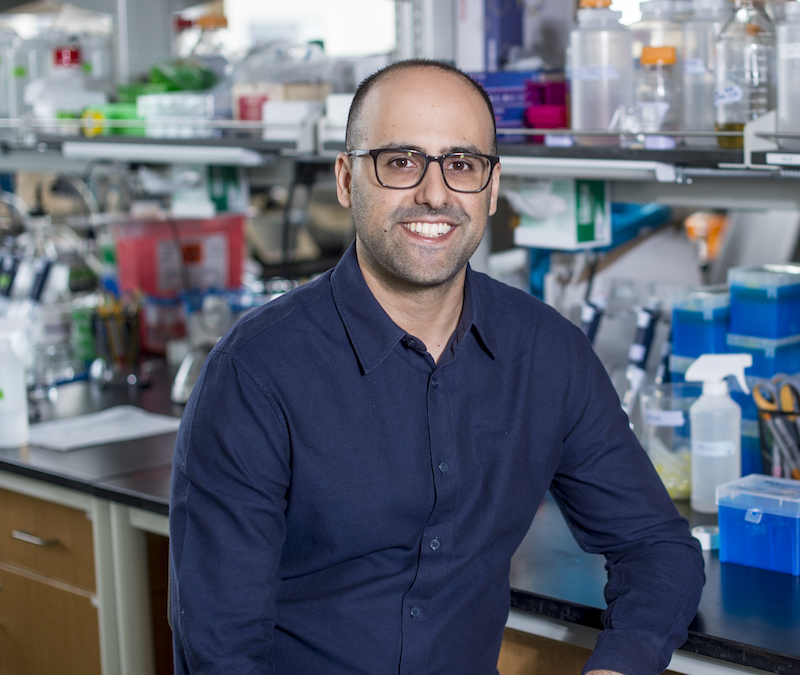
We spoke with Dr. Danino recently to learn more about the research he is pursuing as part of his CRI Lloyd J. Old STAR grant.
Arthur N. Brodsky, Ph.D.:
Your work is focused on using bacteria to improve how we treat cancer, which fits in well with CRI’s origins. Several people central to CRI’s history made cancer breakthroughs in this area, starting with Dr. William B. Coley, who is known as the Father of Cancer Immunotherapy and whose daughter, Helen, founded CRI, and continuing with Dr. Lloyd J. Old.
Many people might not be aware that bacteria have anything to do with cancer, let alone that bacteria can be modified to have anti-cancer properties. What led you to pursue this approach?
Tal Danino, Ph.D.:
There was a confluence of factors as well as digging through the right place at the right time. There are three fields that fundamentally fit into this new approach of engineering bacteria as a cancer therapy.
The first field is microbiology, which recently has changed not only our science but our culture. Indeed, we now understand that bacteria can be good as well as bad. There are thousands of different species of bacteria in the human body and collectively, along with viruses, fungi, and other microscopic organisms, they form the microbiome. Importantly, these bacteria interact with our cells and are needed to help the body carry out many important functions. The impact of microbiology within our research has equipped us with the necessary tools to discover, characterize, and understand the roles that bacteria play in human health. This could potentially build on the idea that we could change our microbiome through diet or with probiotics for not only improving health but also curing diseases such as cancer. Thus, the concept of using bacteria as a therapy has challenged the scientific dogma of how they were viewed as “all bad” over the past decades – even after 100 years ago during the time of William Coley, when he was the first to use bacteria to treat inoperable sarcomas, an aggressive form of cancer that grow in connective tissue like bones, nerves, muscles, tendons, cartilage, and blood vessels. Not only are bacteria found throughout our bodies, but they’re also found inside people’s tumors, and scientists are now sequencing tumors to characterize these bacteria to determine what roles they play during cancer development.
The second important field is cancer immunotherapy. As mentioned earlier, Dr. Coley was the pioneer of using bacteria in the first cancer immunotherapies. And CRI, founded by Coley’s daughter, Helen, is an amazing organization that recognized early on that this field would be an important part of treating and hopefully curing cancer. With the 2018 Nobel Prize being awarded to Dr. James Allison, the director of the CRI Scientific Advisory Council, cancer immunotherapy has now been cemented as the fourth pillar of cancer treatment.
The third field, which has been most important in terms of where I originated, is synthetic biology. In particular, using synthetic biology to engineer living cells and bacteria. To give you my personal story—growing up I was always excited about technology and science. I loved playing with old computers and just messing around with them. I got really interested in science, mostly in physics, math, and chemistry. But as I started studying biology in college, it became a super exciting field because people started to utilize technology to study biological systems, like cancer or bacteria. I did my Ph.D. in synthetic biology, trying to learn how to re-program bacteria and build what we call genetic circuits that could control their behavior, both of individual bacteria and of bacterial communities. As things went along, I wanted to focus more on the potential applications for patients, and I started to think about how we could merge our knowledge of bacteria and our ability to program them in ways that enable us to diagnose or even treat cancer. When I started to look deeper into this during my postdoc, obviously the work of William Coley came up as well as the work of Lloyd Old, who showed that bacteria could be used to treat bladder cancer. Their research actually contributed to the first FDA-approved immunotherapy in 1990, which uses a weakened strain of bacteria.
Arthur N. Brodsky, Ph.D.:
It’s incredible to think how little was known about the immune system in Coley’s time, and to some extent early in Lloyd Old’s career. From what I understand, Coley didn’t even realize that his treatment likely worked because the bacteria triggered the activity of the immune system against tumors; he thought it was just the bacteria themselves attacking tumors.
What is our current state of understanding with respect how bacteria naturally interact with our immune system, and how bacteria can influence the effectiveness of treatments against cancer?
Tal Danino, Ph.D.:
You’re right, it is incredible to think about how little they knew back then. And yet they still had success in some patients. Our understanding has definitely come a long way, but it’s still not completely fleshed out.
Traditionally, when we’ve thought about bacteria from a therapeutic perspective, we think of how bacteria can negatively impact us. We think about bacteria getting into the bloodstream and infecting us or possibly causing a damaging immune overreaction known as cytokine storm. All of these can lead to sceptic shock and organ failure. We are all aware that bacteria being in the wrong place inside the body is very dangerous.
There’s one component to how our immune system interacts with bacteria that’s really unique in the context of cancer, which is that the cores of many tumors are immuno-privileged. What that means is that the immune system doesn’t really have much access to the inner regions of the tumor. Essentially, bacteria can use the core as a safe haven to hide. If they were anywhere else, especially in the blood, they would be eliminated.
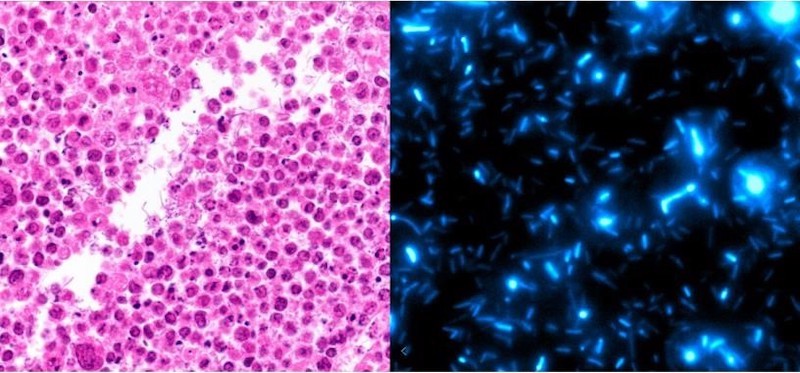
Another place that harbors a lot of bacteria in a unique fashion is the gut. Our intestinal tract is home to a lot of diverse bacteria, many beneficial and necessary. In addition to helping us digest food, these bacteria interact with and help educate the immune system in complex ways. They can also impact cancer and how it responds to treatment, both with chemotherapy and immunotherapy.
Arthur N. Brodsky, Ph.D.:
The fact that bacteria in your gut can affect how melanoma on the skin responds to treatment is such a fascinating concept, it reminds me of Einstein’s “spooky” action at a distance phenomenon in quantum physics.
As you’ve been discussing, there’s a long history behind our current knowledge of how bacteria naturally interact with our immune system and how that impacts how we treat cancer. Now, you want to take it a step further and customize bacteria to make them better at stimulating the immune system to go after cancer.
You mentioned using gene circuits to reprogram bacteria. Could you explain what you mean by gene circuits, and then discuss some of the strategies you’ll be exploring?
Tal Danino, Ph.D.:
The history of synthetic biology is interesting because we’ve been using recombinant DNA and genetic engineering technologies in bacteria since the 1970s to produce different kinds of human proteins, such as insulin for people with diabetes.
But for a while, genetic engineering didn’t incorporate a lot of what we think about as the engineering approach, which revolves around the concept of design-build-test. It’s a cyclical process where you design systems, you try to build them and then test them, and then that leads you to the next design iteration, where you can build off what worked and get rid of what didn’t.
A lot of how we design these systems revolves around mathematical models, so it’s not always simple and trivial. Whether you want to build a toggle switch, something where you just dose it once and then it stays on forever, or something like an oscillator, which goes on and off, you have to figure out how you would rearrange some genes to actually do that. In biology and many molecular systems, a lot of organisms already do that naturally.
So, this is what the field of synthetic biology is really about now, going beyond just trying to make a certain protein to starting to design what we call genetic circuits. These will be collections of genes that are interacting with one another to produce a particular kind of dynamic behavior. The importance of that in the context of human health and therapeutics specifically is this idea of being able to “sense and respond.” Indeed, when we think about what we want our next generation therapies to do, we want them to go beyond chemotherapy, which just acts everywhere, and even other drug delivery vehicles like nanoparticles, which can sense and respond maybe once but are not engineered living medicines that can do this multiple times over a long period.
This sense and respond concept requires using synthetic biology to build a genetic circuit, such as within the genome of a bacterium. Then, one component of the circuit is designed to sense whether or not it's in a tumor environment. If—and only if—it senses that it is within a tumor, it triggers another component of the circuit to start to make a drug or do other things that would be detrimental to the tumor and beneficial for the patient.
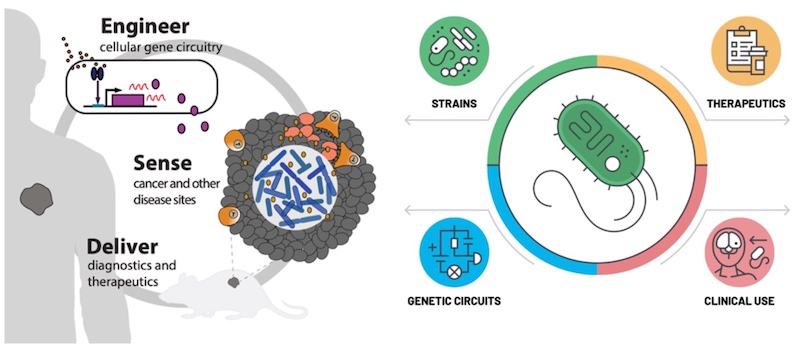
Beyond sense and respond, I think the new paradigm is trying to engineer living medicines, similar to CAR T cells and other cell therapy-based approaches. It’s interesting to compare them to bacteria, because whereas CAR T cells have not had much success when it comes to infiltrating and attacking solid tumors, bacteria readily penetrate these cancers. In this regard, I think they have a different role as a therapeutic modality. As I alluded to in my proposal for this CRI project, the future is not just going to be focused on bacteria, but on how you can get all these different modalities to start interacting with and synergizing with one another.
Arthur N. Brodsky, Ph.D.:
That makes sense. Even with how effective immunotherapy is in some types of advanced cancer, it still doesn’t work for the majority of patients, and it’s going to take different combinations of approaches to cure everyone.
In your STAR application, you described using engineered bacteria as ‘trojan horses’ to infiltrate and undermine tumors from within. Once there, they can deliver drugs, such as chemotherapies that stop the tumor from growing, or immunotherapies that boost the immune system. How could this approach enhance the effectiveness of existing treatments, especially immunotherapies?
Tal Danino, Ph.D.:
That’s a good question. The coolest part about the approach is that bacteria colonize solid tumors regardless of their genetics. This is the key that differentiates this approach from other technologies. Being able to penetrate the low-oxygen, immune-privileged cores of solid tumors is huge, because solid tumors account for more than 90% of cancer-related cases and deaths.
This really unique feature allows us to get in “for free” in a way, without having to engineer that capability into the bacteria. They can colonize the tumor core, the deepest and most difficult region to target with other types of drugs. Bacteria can act on the deepest parts of the tumor, and you can have other drugs act on the proliferating outer parts of the tumor. For this reason alone, bacteria are a really interesting modality to combine with other therapies.
Regarding how we could specifically engineer them, we are thinking about how we could improve specificity, safety, and efficacy. From the safety perspective, we only want the bacteria to make the drug and to grow within the tumor, so we’re building several kinds of sensor machinery that could enable the bacteria to clearly distinguish a tumor from healthy tissue.
With these bacteria making a drug, you have to think about how you are going to get that drug to act on the cancer cells or the immune cells inside the tumor. We’re still exploring several strategies here, and we’re thinking about what types of therapeutic cargo we should make that would be potent at helping to eliminate tumors, whether directly or by engaging the immune system. There are so many things that we could make. To explore these different possibilities, we’re using an artificial tumor system, called spheroids, to screen many different cancer-killing drugs that we could use as cargo.
When it comes to immunotherapy cargos, it’s hard to test those in the lab in such a system. Therefore, we’re looking at therapeutics that other people have been developing or have been tested in clinical trials. Sometimes these drugs can have toxicity when they’re delivered systematically, so by delivering them only within the tumor we hope to alleviate some of the immune-related adverse effects while also being able to achieve higher local doses within the tumor.
Arthur N. Brodsky, Ph.D.:
I'm heartened to hear about all the potential applications of these bacteria-based therapies.
Now, I want to bring up PD-1/PD-L1 checkpoint immunotherapies, which are probably the most broadly successful immunotherapies today. These treatments work by preventing tumors from shutting down ongoing immune responses, and they’re most effective against tumors that have already been infiltrated by T cells that recognize the cancer cells. But in most cases, tumors either exclude these T cells or lack them altogether, and this renders PD-1 immunotherapy ineffective.
For these hard-to-treat tumors, could you potentially engineer bacteria to produce molecules that attract the immune system, and thereby trigger immune responses from scratch and enhance the power of immunotherapy?
Tal Danino, Ph.D.:
Exactly. That’s something that we’re very interested in. We’ve shown that a cytokine called GM-CSF can recruit T cells and other immune cells. And in “colder” tumors that are lacking in immune cells, it had a stronger effect in combination with checkpoint immunotherapy molecules produced by the bacteria. So those together was more effective than one or the other, exactly like you’re saying.
Okay I think that’s the right strategy, so we’re still thinking about what cells we should recruit and what kinds of molecules are available that we could use to do that. While cytokines and chemokines can accomplish that, they have other effects that we don’t necessarily want. For example, you could recruit some “killer” T cells but you might also be recruiting regulatory T cells, which can suppress anti-cancer immune responses. The way we are trying to think about it is: what could we make that will recruit the right cells and push away the wrong cells? What are the combinations of things that we should make to end up with the right immune balance within the tumor?
Arthur N. Brodsky, Ph.D.:
As you mentioned, there’s still just a lot of basic biology to learn about how bacteria in our bodies and the immune system interact. And then with respect to the engineering side of it, there are a number of different strategies you could pursue, a number of different molecules and pathways to try to target with your engineered bacteria.
As you pursue these questions and explore various approaches, how important is the CRI STAR funding for you, especially with the long-term flexibility it provides to follow the science where it leads you?
Tal Danino, Ph.D.:
Fundamentally understanding the interactions between bacteria and the immune system in the context of cancer is extremely important and will help lead us to better therapies. It’s something that we definitely want to pursue and previously didn’t have funding for. Having the flexibility to pursue that at the same time as we optimize our engineering and translational process is extremely valuable.
I think that CRI is the perfect institution for this type of work because a lot of the history is tied to Drs. Coley and Old and these fundamental concepts of trying to understand immunology better in order to inform new therapies. I actually live a block away from the old New York hospital where Coley worked. It’s kind of fun to walk by it—it makes me think about all the things he did to lay the foundation that helped us get to where we are now. I had read so much about him in the past, and this funding from CRI is the perfect award for this kind of approach.
Arthur N. Brodsky, Ph.D.:
What do you hope you’ll be able to accomplish after five years as a CRI Lloyd J. Old STAR? And how do you hope your work will impact the field of cancer immunotherapy?
Tal Danino, Ph.D.:
I would like bacteria-based therapies to be seen as a new modality in the cancer immunotherapy arsenal. Over time, I think they will be. There’s probably about a dozen groups working in preclinical and clinical development of bacteria therapies right now. We’re at the start of what should be a really exciting revolution in these new bacteria-based immunotherapies. They’re so easy to engineer and they’re everywhere in our bodies and they're related to so many diseases that I think it’s inevitable that people will focus more and more on bacterial therapies moving forward.
Specifically, with respect to our work, you hit upon something really important—we’re really trying to better understand bacteria in the context of cancer, so that we can engineer them to be better immunotherapies. We hope that we can identify some promising bacterial therapy candidates through our translational studies so that we could then advance to clinical trials. We’re already starting to do that, but I think with this award we will be able to develop new candidates with even better effectiveness.